Columbia University
Irving Medical Center
Neurological Institute
710 West 168th Street, 3rd floor
(212) 305-1818


TaubCONNECT Research Perspective:
August 2023
2: Multicellular Communities are Perturbed in the Aging Human Brain and Alzheimer's Disease
3: Simple Topological Task-based Functional Connectivity Features Predict Longitudinal Behavioral Change of Fluid Reasoning in the RANN Cohort
4: The Neuropathological Landscape of Hispanic and non-Hispanic White Decedents with Alzheimer Disease
![]() | ![]() | ![]() | ||
Richard Mayeux, MD, MSc | Nilüfer Ertekin-Taner, MD, PhD | Caghan Kizil, PhD |
An early consequence of Alzheimer's disease (AD) pathology manifests as a diminished capacity for generating new neurons. The restoration of neurogenesis stands as a potential therapeutic avenue, capable of ameliorating the compromised resilience of the brain in this context. However, the mechanisms underlying the reversal of pathology-induced impairment in neurogenic potential remain unknown.
To understand how neurogenesis can be increased after AD in mammalian brains, we studied zebrafish brain, which is a remarkable regenerative organ where AD pathology leads to significant neurogenesis from astroglia, the neural progenitors. This regenerative response pivots on the activity of the astroglial nerve growth factor receptor (Ngfr). Notably, Ngfr is absent in mammalian astroglia, potentially contributing to the compromised neurogenic competence seen in AD. Within the scope of this study, now published in npj regenerative medicine, we activated Ngfr in astroglia within an AD mouse model. This intervention prompted a transition of these cells from a reactive state, typically associated with gliotic scarring, to states conducive to neurogenesis. This transformation culminated in the generation and integration of new neurons throughout the hippocampus. Intriguingly, the amplified neurogenesis exerted a mitigating influence on characteristic AD pathological hallmarks—amyloid plaques and the advanced phosphorylation of Tau protein. Furthermore, we found a molecular switching module dictating the conversion of reactive astroglia into their neurogenic counterparts. In human AD cohorts, we noted elevated levels of Lipocalin-2 (LCN2) and Phosphofructokinase (PFKP). In mice, we determined that Lipocalin-2 (Lcn2), a recognized marker of reactive astroglia, operates through its receptor Slc22a17 to potentially activate Pfkp. In vivo (utilizing mouse brains) and in vitro (employing a 3D hydrogel-based primary human neurogenesis assay), interventions that blocked these factors augmented the neurogenic potential of mammalian astroglia.
Figure. (1) LCN2 (purple) is upregulated in reactive astroglia (green) in human brains with AD. (2) Stable expression of NGFR (red) in a few mouse astrocytes leads to significant newborn neurons (red and green) in the hippocampus. (3) NGFR expression (red) reduces amyloid burden (green) and Tau hyperphosphorylation (not shown). (4) Synopsis of the
molecular mechanism of how neural progenitors can toggle between reactive and neurogenic states in AD.
|
This study not only underscores the centrality of neurogenesis in its interplay with the mitigation of AD pathology but also reveals new potential pharmaceutical targets, notably SLC22A17 and PFKP. Serving as an exemplar of the "zebrafish-to-human" approach, this study proposes therapeutic avenues by harnessing the innate regenerative capabilities of vertebrate brains to counterbalance AD pathology and enhance neural resilience.
Caghan Kizil, PhD
Associate Professor of Neurological Sciences (in Neurology and in the Taub Institute)
ck2893@cumc.columbia.edu

Multicellular Communities are Perturbed in the Aging Human Brain and Alzheimer's Disease
![]() | ![]() | ![]() | ||
Mariko F. Taga, PhD | Vilas Menon, PhD | Philip L. De Jager, MD, PhD |
A new study from our Center for Translational and Computational Neuroimmunology aimed to address several key questions regarding the role of different cell types and their potential interactions in Alzheimer’s disease (AD). In collaboration with co-authors Naomi Habib and Anael Cain from The Hebrew University of Jerusalem and other colleagues, we assembled a high-resolution cellular map of the aging frontal cortex using single-nucleus RNA-sequencing of 24 well-characterized individuals, integrated with bulk RNA-sequencing profiles from the same brain region in a larger set of 638 individuals. All participants were part of longitudinal studies on cognitive aging, with detailed assessments and prospective autopsy data.
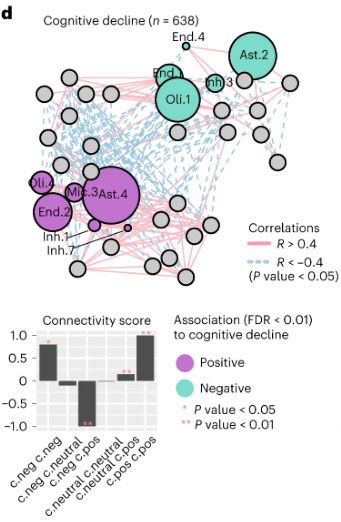
Figure 6d. Cellular communities are linked to AD-associated traits. Cellular network of coordinated and anti-coordinated cell subsets (nodes), colored by the associations (multivariable linear regression, FDR 0.01) with AD traits (purple, positive; green, negative association; gray, nonsignificant) for cognitive decline.
As recently reported in Nature Neuroscience, this novel approach combining single-cell and bulk molecular profiling approaches enabled the identification of specific subpopulations of cells associated with AD pathophysiology, including specific subtypes of oligodendrocyte and microglial cells, as well a decrease in inhibitory neurons expressing somatostatin. Our study also uncovered a network of coordinated multicellular communities in the human neocortex; these cellular communities are composed of different cell subtypes which co-occur among individual. One of these cellular communities is associated with ante-mortem cognitive decline and post-mortem tau pathology burden. Thus, the cellular target in Alzheimer’s disease is not a single cell type but a set of different cell subtypes (astrocytes, microglia, oligodendrocytes,..) that increase in frequency over the course of the disease in a coordinated fashion and are communicating with one another.
Disrupting these pathology-associated cellular interactions may be an approach with which to prevent cognitive decline related to Alzheimer’s disease. By identifying cellular factors contributing to cognitive decline and AD, this project also provides insights that can guide future cell type-specific investigations, particularly of oligodendrocyte populations whose role in AD remains poorly understood. Overall, the integration of detailed single-nucleus profiles with a deeply phenotyped large-scale cohort enhances our understanding of the cellular landscape in Alzheimer’s disease and its implications in the disease.
Mariko F. Taga, PhD
Assistant Professor of Neurological Sciences (in Neurology and in the Taub Institute)
mt3203@cumc.columbia.edu
Vilas Menon, PhD
Assistant Professor of Neurological Sciences (in Neurology and the Taub Institute)
vm2545@cumc.columbia.edu
Philip L. De Jager, MD, PhD
Weil-Granat Professor of Neurology (in Neurology and the Taub Institute)
pld2115@cumc.columbia.edu

![]() | ![]() | |
Georgette Argiris, PhD | Christian Habeck, PhD |
Neuroimaging techniques have revealed synchronous patterns of neural activity between functionally-related regions in the brain, which has typically been operationalized by Pearson’s correlation to capture the statistical dependency (i.e., edges) between temporal signals arising from anatomically distinct brain regions (i.e., nodes). Functional connectivity (FC) measured at rest has revealed that when these correlations are computed across multiple areas, the brain forms a complex large-scale network organization with modular architecture, where high segregation between modules, or systems, supports healthy brain function. Research in our lab and others has shown that aging is typically linked to a decrease in segregation of functional brain systems. Due to the complex structure and graph-like behavior of brain connectivity, more recent attention has been given to topological data analysis (TDA), and more specifically persistent homology (PH), to identify the underlying shape of brain network connectivity beyond simple node pairings by computing connective components and other low-dimensional features of a network across different Pearson correlation thresholds.
Figure 1. Left panel: Hypothetical Betti-0 curve for toy example of 18 nodes (n) in a brain network across five filtration thresholds (ε). When ε =0, B0 is equal to the number of nodes in the network (=18), as each isolated node is a component in itself. When ε =0.2, all nodes are linked via at least one connection, forming a single component and thus yielding a B0 of 1. The B0 curve is characterized in terms of the area under the curve (AUC), shaded in purple. Analysis is performed at the participant-level. Right panel: Scatterplot of the relationship between baseline Age, longitudinal change in AUC (ΔAUC), and longitudinal change in cognition (Δ Cognition) for Fluid Reasoning. Each dot represents a participant and the color depicts the age band to which the participant belongs. The gray line represents the least-squares fit.
|
In the current work, we used the simplest measure from PH— dimension 0 capturing the number of connected components— to analyze brain network connectivity between 264 selected brain regions. Our goal was to understand how these functional connections change with age and how this affects cognition. We examined longitudinal data at two time points from 163 participants aged 20 to 80 years who underwent both brain scans and in-scanner cognitive testing in four domains of cognition. We focused on a measure called the area under the Betti-0 curve (=AUC), which considers the number of components formed between connected nodes as the network is incrementally pruned at correlation thresholds in descending order of connection strength; here, the correlation thresholds were determined by edge density, or an incremental increase of one percentile in the correlation distribution. Low AUC values indicates that the 264 brain regions express a network structure with overall fewer connected components, as a single connected component is quickly formed over few pruning thresholds. As recently reported in Neuroimage, we found that the AUC decreased over time with advancing age in the cognitive domain of Fluid Reasoning. This decline in AUC was associated with declines in cognitive performance beyond factors like age, demographics, and brain health. The changes in AUC partially explained the effects of aging on cognitive decline. Additionally, we observed that, while systems segregation decreased with age in three of the four cognitive domains, it did not account for changes in cognitive performance in any domain. These findings highlight the usefulness of using PH and similar methods to better understand the aging brain and its impact on cognitive abilities.
Georgette Argiris, PhD
Postdoctoral Research Scientist in the Gertrude H. Sergievsky Center
gla2116@cumc.columbia.edu
Christian Habeck, PhD
Professor of Neurological Sciences (in Neurology and the Taub Institute) at CUMC
ch629@cumc.columbia.edu

The Neuropathological Landscape of Hispanic and non-Hispanic White Decedents with Alzheimer Disease
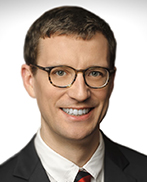
Andrew F. Teich, MD, PhD
Diversity is a major issue for brain tissue repositories in the United States. Most post-mortem CNS tissue collections are primarily from Caucasian subjects, and thus do not reflect the diversity of the US population. This lack of diversity has implications for research using these materials, as conclusions that are drawn from this work may not be as applicable to under-represented groups. In Scalco et al., published in Acta Neuropathologica Communications, our investigative group made an effort to address this issue in one specific area by comparing Alzheimer’s disease (AD) neuropathology in non-Hispanic White decedents (NHWD) to Hispanic decedents (HD). In order to assemble a cohort with maximal statistical power, we collected tissue from three ADRC sites – UC Davis (where the lead investigator, Dr. Brittany Dugger, is based), Columbia University, and UC San Diego. This yielded a collection of 92 HD samples and 185 age and sex matched NHWD samples, and all subjects had intermediate to high AD neuropathologic change.
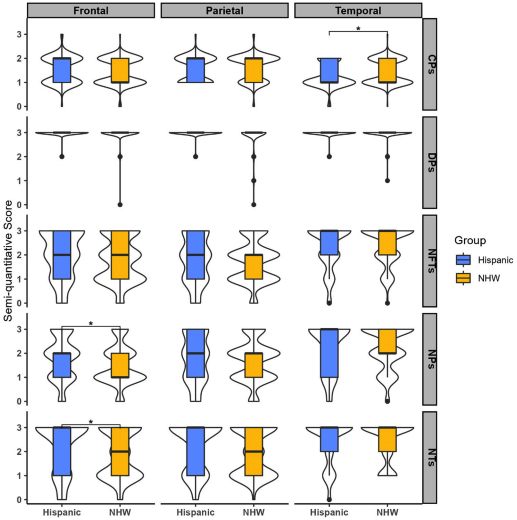
Figure 2. A visualization comprising of a combination of violin and box plots was used to depict the distribution of various pathologies (cored plaques: CPs, difuse plaques: DPs, neuritic plaques: NPs, neuropil threads: NTs, and neurofbrillary tangles: NFTs) in three specifc brain areas-frontal, temporal, and parietal cortices. The violin plots were used to display the distribution of the data, while the boxes indicate the range between the frst and third quartiles. The bold horizontal line inside the boxes represents the median value. Additionally, the whiskers extend beyond the upper and lower limits of the box and indicate the range of data within 1.5 times the length of the box.
Comparison of these cohorts across four areas (posterior hippocampus, frontal, temporal, and parietal cortices) demonstrated that HD subjects had statistically higher neuritic plaques and neuropil threads in frontal cortex, while NWHD had higher levels of cored plaques in temporal cortex. Although preliminary, this suggests that frontal cortex pathology may be more pronounced in HD subjects with AD neuropathologic change. Limitations include the relatively small sample size, which is exacerbated by heterogeneity in the HD group (which drew from multiple cohorts, with Mexico, Puerto Rico, the Dominican Republic, Cuba, and South America included as ancestral regions). Additionally, the reason for the observed discrepancy is unclear, and a variety of socioeconomic and environmental factors could be considered. Nevertheless, this initial study demonstrates the importance of examining AD pathology in a multi-ethnic cohort, and will motivate larger studies to further clarify how AD pathology develops in different populations. In addition, these findings demonstrate the importance of community outreach to enhance the diversity of tissue repositories, which will help to make AD research reflect the diversity of our population.
Andrew F. Teich, MD, PhD
Associate Professor of Pathology and Cell Biology (in Neurology)
aft25@cumc.columbia.edu
