Columbia University
Irving Medical Center
Neurological Institute
710 West 168th Street, 3rd floor
(212) 305-1818


TaubCONNECT Research Perspectives:
March 2015
neuroinflammation measured with PET radioligand binding to translocator protein (TSPO)
» #2 Assembly and Interrogation of Alzheimer's Disease Genetic Networks Reveal Novel
Regulators of Progression
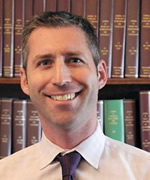
William Charles Kreisl, MD
Chronic inflammatory change in the brain may play an important pathogenic role in neurodegenerative disorders such as Alzheimer's disease (AD). The 18 kDa translocator protein (TSPO) is over-expressed by activated microglia and reactive astrocytes and, therefore, has been used as a biomarker for inflammation. Positron emission tomography (PET) can quantify inflammation in vivo using radioligands that bind to TSPO. In prior studies, Taub faculty member Dr. William Charles Kreisl has used one such radioligand, 11C-PBR28, to demonstrate that TSPO binding correlates with severity of Alzheimer's disease. While these results suggest that 11C-PBR28 may be useful in monitoring disease progression or response to treatment, the standard methods of 11C-PBR28 PET require arterial blood sampling and 90 minutes of scan time.
As a less invasive alternative method of 11C-PBR28 PET, Dr. Kreisl and members of the National Institutes of Health Molecular Imaging Branch and the National Institute of Mental Health used the cerebellum as a pseudo-reference region to create regional standardized uptake value ratios (SUVR), recently published in The Journal of Nuclear Medicine. The cerebellum was chosen because it is relatively free of neurodegenerative changes early in AD and results from prior studies showed no difference in 11C-PBR28 binding in cerebellum between AD patients and controls. These SUVR values were compared to those obtained from the "gold standard" method of 11C-PBR28 measurement. Dr. Kreisl's team determined that SUVR measurement distinguished AD patients from controls in more regions than the gold standard measurement and with lower variability. The SUVR method also shortened the amount of time subjects were required to be in the scanner, because only 30 minutes of scan data (taken from 60 to 90 minutes after injection of 11C-PBR28) were needed to distinguish subject groups. Moreover, SUVR values correlated with clinical severity as measured using the Clinical Dementia Rating scale.
Dr. Kreisl and his team concluded that SUVR measurement of 11C-PBR28 binding was as good, if not superior, to the gold standard measurement, while avoiding need for arterial catheterization and requiring shorter scan time. Therefore, using SUVR for 11C-PBR28 PET imaging is expected to improve subject tolerability and statistical power in future studies of patients with Alzheimer's disease.
William Charles Kreisl, MD
Assistant Professor of Neurology (in the Taub Institute)
wck2107@columbia.edu

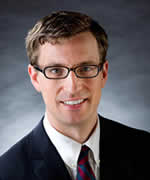
Commentary provided by Andrew Teich, MD, PhD, Assistant Professor of Pathology and Cell Biology (in the Taub Institute)
In this recently published PLOS ONE paper, Aubry et al. investigate disruption of gene expression in Alzheimer's disease (AD) using a novel computational biology approach. To understand the importance of this paper, it helps to have some historical perspective. From a therapeutic point of view, the AD field has been characterized by serial disappointment. The two proteins that famously aggregate in AD brain (beta-amyloid and tau) have been the focus of immense study. Therapeutic approaches to AD have largely focused on clearing beta-amyloid from the brains of AD patients, but this approach has failed so far. Although clearance of amyloid may ultimately prove useful, and tau-clearing approaches may be successful, the lack of therapeutic progress should encourage new approaches to investigating the pathogenesis of AD.
The Aubry et al. study utilizes a data set from the Banner Alzheimer's Institute that consists of DNA expression data from 58 brains with varying stages of AD pathology (ranging from normal to advanced AD). Each brain was also sampled in up to six different regions, yielding 193 useable expression profiles. The regions sampled ranged from areas affected early in AD (such as hippocampus) to areas affected late or not at all (visual cortex). Thus, the 193 expression profiles cover a range of disease stages, both at the subject and brain regional level. With this large number of expression profiles, Aubry et al. were able to take advantage of a set of computational techniques that allow one to determine the relationship between expression of a transcription factor and a downstream target. These tools assume that if a transcription factor drives the expression of a target gene, then if the transcription factor's mRNA changes, there should be a correlation with the mRNA of the downstream target. There are many intervening steps that will make this relationship statistically weak, such as translation, protein turnover rates, etc. Thus, to capture this statistical relationship, many independent data sets are required; the 193 number in this paper is adequate for this analysis. Using this approach, Aubry et al. map out a large number of putative interactions between transcription factors and downstream targets, and they then use this relationship to predict which transcription factors have impaired activity in AD. This is done by looking at the entire data set, and asking if all of a given transcription factor's proposed target mRNAs are changing in AD in a way that would be consistent with increased or decreased activity of said transcription factor.
One of the biggest flaws in computational biology work is that investigators sometimes do not test their own predictions. Theoretical papers can remain entirely theoretical, and this invites analysis that sometimes looses biological focus. One of the biggest strengths of the Aubry et al. paper is that it biochemically validates the top three transcription factors that it predicts to be dysregulated in AD. These results also shed light on possible mechanisms of dysregulated acetylation of histones and other signaling proteins, such as p53. These results are not only interesting in their own right, but they validate the overall approach of Aubry et al. Finally, these results are not mutually exclusive from the view that amyloid and/or tau are central to the pathogenesis of AD. However, simply because a protein is pathogenic does not necessarily mean that it is a drugable target, or represents the best therapeutic approach. The unbiased, computational approach of Aubry et al. not only has the opportunity to shed further light on how amyloid and/or tau contribute to AD pathogenesis, but may also suggest novel therapeutic targets.
Corresponding Authors
Andrea Califano
Clyde and Helen Wu Professor of Chemical Systems Biology
Chair, Department of Systems Biology
Director, JP Sulzberger Columbia Genome Center
Associate Director, Herbert Irving Comprehensive Cancer Center
ac2248@columbia.edu
Michael Shelanski
Delafield Professor of Pathology and Cell Biology (in Neuroscience and in the Taub Institute for Research on Alzheimer's Disease and the Aging Brain)
Chair, Pathology and Cell Biology
Pathologist-in-Chief, NewYork-Presbyterian Hospital/Columbia University Medical Center
Co-Director, Taub Institute for Research on Alzheimer's Disease and the Aging Brain
Director, Medical Scientist Training Program
mls7@columbia.edu
