Columbia University
Irving Medical Center
Neurological Institute
710 West 168th Street, 3rd floor
(212) 305-1818


Featured Research
In the Lab:
Abid Hussaini, PhD
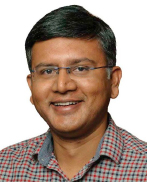
Abid Hussaini, PhD
We are interested in how memories are made and lost in neurodegenerative diseases, such as Alzheimer’s disease (AD). Most of us are able to faithfully store a mental picture (cognitive map) of our surroundings, but this becomes increasingly difficult for those with dementia. Problems with spatial memory are common in dementia with symptoms such as disorientation and getting lost frequently even in familiar environments, appearing early in the disease. To understand what causes spatial memory dysfunction, we are using mouse models of AD and investigating the brain regions important for spatial memory—the hippocampus and the entorhinal cortex. My lab specializes in electrophysiological techniques to record the activity of neurons in freely behaving animals. This allows us to evaluate the function of the brain. Our aim is to determine how neurons in the EC-HPC (entorhinal cortex and hippocampal) circuit function in the presence of amyloid beta, tau, or both.
The neurons in the EC-HPC circuit are well characterized and have unique spatial properties. The pyramidal neuron of the hippocampus-place cell, fires an action potential at a specific location of the animal and is termed a “place field.” Multiple place fields make up an animal's “mental map” allowing us to assess spatial memory accurately. We found that in the hAPP/J20 mice (with amyloid beta pathology) the place cells fire less precisely, which indicates that the representation of space in these animals is distorted (Fig.1). The pyramidal and stellate cells of the medial entorhinal cortex (MEC) have unique cells types too. The grid cells, for example, fire symmetrically in a grid-like triangular pattern throughout the environment of the animal. It is known to be important for path integration—the ability to make a shortcut while navigating. The MEC also has head-direction cells, which fire exclusively in the direction the animal is facing, and the border cells, which fire when the animal displays thigmotaxis (walking along the walls) (Fig.2). Our experiments with hAPP/J20 mice reveal that presence of amyloid beta increased the overall neuronal firing. We have yet to determine if this affects all cell types or specific ones. In a recent paper, we showed that tau pathology in EC (EC-Tau) affected only the grid cell function, while sparing head-direction cells and border cells. The firing rate of the grid cells reduced and the pattern of neuronal firing became less grid-like, indicating a loss of function. The overall network activity was altered, too, with only theta frequency oscillations affected and other frequencies spared in the tau pathology mice. The EC-Tau mice were impaired in T-maze, Morris water maze, and novel object recognition task, indicating that some of the downstream hippocampal regions are affected.
Place Cell Dysfunction |
Grid Cell Dysfunction |
|
![]() Figure 1. Place fields in the hAPP/J20 mice are less precise. Place cell firing rate maps for 6 month old control (top), 30 month old control (middle) and 18 month old hAPP/J20 (bottom) mice recorded as they freely explored a 50 x 50 cm square box for 15 min. Place fields in 30-mo old control mice appear stretched compared to 6-mo old controls. While place fields in 18-mo old hAPP/J20 mice appear fuzzy and less precise indicating place cell dysfunction resulting from amyloid beta pathology. |
![]() Figure 2. Top- Left: Firing of one grid cell (pink dots) after the animal explored the 50cm2 box for 30 minutes. Black lines indicate animal’s running path. Middle: firing rate map of the grid cell firing. A range of blue to red colors indicate low to high firing rate respectively. Black equilateral triangle indicates that the points of highest firing rate of this grid cell are symmetrical. Right: Autocorrelation map of the firing rate map showing symmetrical layout of the grid pattern. Middle- Left: Firing rate and autocorrelation maps of a grid cell in a 30-month old control versus EC-Tau mouse. The grid cell firing map in the EC-Tau mouse appears less symmetrical compared to the control indicating grid cell dysfunction. Bottom- Left: Tuning of head direction cell in a 30 month old control vs EC-Tau mouse shows no difference. The rose plots are mostly tuned around NW direction in control animal and N direction in EC-Tau mice. Right: Border cells fire very close to the edge of the wall in control (East) and EC-Tau (South) mouse with no significant deviations from the edge. Courtesy: Dr. Gustavo Rodriguez |
Optogenetic Stimulation of Neurons
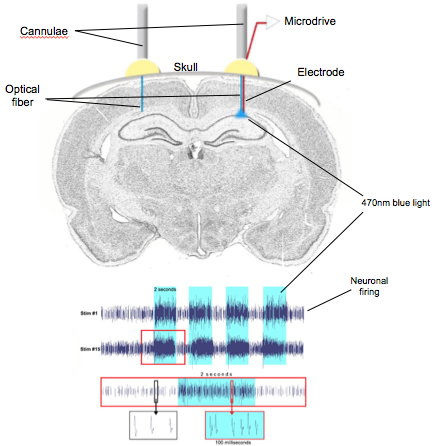
Figure 3. Cartoon showing the in vivo experimental setup and results. Top- Metal cannulae implanted on the mouse skull with optical fibers in the left and right hemispheres of the brain to optically stimulate channelrhodopsin (ChR2) expressing neurons with 470nm blue light. The right hemisphere is implanted with a microdrive carrying 16-channel electrodes to record neuronal activity from the hippocampus. Bottom- Extracellular recording from the neurons of the hippocampus showing increased firing activity with blue light (470nm). Rows 1 (stimulation #1) and 2 (stimulation #15) showing four pulses (blue bars) of 2-second stimulation at 30Hz frequency increasing the firing activity of the hippocampal neurons. Third row is a zoom-in of the top row (red inset) showing neuronal firing with (blue bar) and without the blue light. Bottom row showing the spike firing rate and the waveform of the neurons in stimulated (green inset) vs unstimulated (black inset) state. The spike firing rate roughly doubled when stimulated with the blue light. .
In parallel with the aforementioned studies, we are using optogenetic and chemogenetic approaches to manipulate the activity of the neurons. In collaboration with Dr. Karen Duff, we have shown that increasing neuronal activity with channelrhodopsin (ChR2) or hM3D (Gq) led to an increase in tau pathology (Fig. 3). We are currently testing multiple optogenes that increase or decrease neuronal firing, to make certain we are able to restore neuronal function without worsening the existing pathology and reverse memory impairments.
Another goal of our lab is to identify behavior tasks relevant to certain brain regions. The MEC, for example, is critical for spatial memory and path integration, while lateral entorhinal cortex (LEC) is important for object recognition and odor discrimination. To evaluate the function of the MEC, we have designed a path integration task in mice. In this, the mouse is trained to navigate an L-maze from its home cage and, on reaching the end of the maze, to return home by taking the shortest route back. Similarly, to identify dysfunction in the LEC, we have the object context recognition (OCR) test that is akin to a novel object test but with multiple contexts. We also have tests to detect olfactory dysfunction, including the odor discrimination task and the nested T-maze task, which help identify if the animal uses the place learning or the response learning strategy to solve the maze.
With multiple regions of the brain affected in AD there is an urgent need to record from these regions simultaneously. To achieve this, we have created a behavior setup where the mouse is head-fixed and navigates a virtual reality maze. This allows us to not only record brain activity from several brain regions simultaneously, but also to evaluate the animal's memory in a variety of complex environments (Fig. 4).
Virtual Reality Setup with Head-fixed Electrophysiology Recording | ||
![]() |
![]() Figure 4. Left- An overall view of the circular path on which the animals will be trained to navigate in virtual reality (VR). Right- Top view of the head-fixed VR setup- the animal is head-fixed to the electrophysiology setup (not shown) and placed on the styrofoam ball (orange with thick black stripe). The VR circular path is displayed onto the two screens in front of the animal. Similar to a joystick in a video game, the rotation of the ball controls the forward or backward movement on the screen giving the perception of motion. It takes about 2-3 days for the animal to learn to navigate the VR environment. The feeding needle (in front of the screen) can be used to motivate the animal with sugar water or milk. Bottom left- Animal’s view of the VR circular path. Movie: Video simulation of the VR circular path. Courtesy: Geoffrey Barrett |
Our collaborators include:
• Dr. Karen Duff: apart from using several novel mouse models for AD, we are collaborating on optogenetic and chemogenetic projects to identify and correct dysfunction due to amyloid beta and tau pathology.
• Dr. Eric Kandel: we are exploring circuit mechanisms that separate age-related memory loss from AD-related dementia.
• Dr. Stefano Fusi: we are using Dr. Fusi's expertise to decode the position of the animal based on the place cell firing patterns.
• Dr. Steve Siegelbaum: place cell encoding in enhanced memory mouse model-HCN1.
• Dr. Elisa Konofagou: we are collaborating on focused ultrasound and optogenetics.
• Dr. Catherine Marquer: we are collaborating on place cell dysfunction in synaptojanin 1 mice.
Altogether, our lab is committed to identifying subtle differences in electrophysiological properties of neurons that will help us diagnose dementia earlier than what is currently known, as well as testing out various optogenetic and chemogenetic approaches in order to correct cognitive deficits.
The Hussaini lab is supported by funding from the NIH (NIA) and Alzheimer's Association.
Abid Hussaini, PhD
Assistant Professor of Pathology and Cell Biology (in the Taub Institute)
sah2149@cumc.columbia.edu
